Assorted Recent Work
Impact of Binary Stars on Planet Statistics — I. Planet Occurrence Rates, Trends with Stellar Mass, and Wide Companions to Hot Jupiter Hosts Moe & Kratter, 2021
more
Close binaries suppress the formation of circumstellar (S-type) planets and therefore significantly bias the inferred planet occurrence rates and statistical trends. After compiling various radial velocity and high-resolution imaging surveys, we determine that binaries with a < 1 au fully suppress S-type planets, binaries with a = 10 au host close planets at 15% the occurrence rate of single stars, and wide binaries with a > 200 au have a negligible effect on close planet formation. We show that F = 43% +/- 7% of solar-type primaries do not host close planets due to suppression by close stellar companions. By removing spectroscopic binaries from their samples, radial velocity surveys for giant planets boost their detection rates by a factor of 1/(1-F) = 1.8 +/- 0.2 compared to transiting surveys. This selection bias fully accounts for the discrepancy in hot Jupiter occurrence rates inferred from these two detection methods. Correcting for both planet suppression by close binaries and transit dilution by wide binaries, the occurrence rate of small planets orbiting single G-dwarfs is 2.1 +/- 0.3 times larger than the rate inferred from all G-dwarfs in the Kepler survey. Additionally, about half (but not all) of the observed increase in small, short-period planets toward low-mass hosts can be explained by the corresponding decrease in the binary fraction. Finally, we demonstrate that the apparent enhancement of wide stellar companions to hot Jupiter hosts is due to multiple selection effects. Very close binaries, brown dwarf companions, and massive planets with M_2 > 7 M_J within a < 0.2 au preferentially have metal-poor hosts and wide tertiary companions, but genuine hot Jupiters with M_p = 0.2-4 M_J that formed via core accretion instead favor metal-rich hosts and do not exhibit a statistically significant excess of wide stellar companions.
MoeKratterI_fig3
The highly variable time evolution of star-forming cores identified with dendrograms Smullen, Kratter et al, 2020
moreWe investigate the time evolution of dense cores identified in molecular cloud simulations using dendrograms, which are a common tool to identify hierarchical structure in simulations and observations of star formation. We develop an algorithm to link dendrogram structures through time using the three-dimensional density field from magnetohydrodynamical simulations, thus creating histories for all dense cores in the domain. We find that the population-wide distributions of core properties are relatively invariant in time, and quantities like the core mass function match with observations. Despite this consistency, an individual core may undergo large (>40 per cent), stochastic variations due to the redefinition of the dendrogram structure between time-steps. This variation occurs independent of environment and stellar content. We identify a population of short-lived (<200 kyr) overdensities masquerading as dense cores that may comprise ∼20 per cent of any time snapshot. Finally, we note the importance of considering the full history of cores when interpreting the origin of the initial mass function; we find that, especially for systems containing multiple stars, the core mass defined by a dendrogram leaf in a snapshot is typically less than the final system stellar mass. This work reinforces that there is no time-stable density contour that defines a star-forming core. The dendrogram itself can induce significant structure variation between time-steps due to small changes in the density field. Thus, one must use caution when comparing dendrograms of regions with different ages or environment properties because differences in dendrogram structure may not come solely from the physical evolution of dense cores. Smullen_2020_fig1
A Disk-driven Resonance as the Origin of High Inclinations of Close-in Planets Petrovich et al 2020
moreThe recent characterization of transiting close-in planets has revealed an intriguing population of sub-Neptunes with highly tilted and even polar orbits relative to their host star’s equator. Any viable theory for the origin of these close-in, polar planets must explain (1) the observed stellar obliquities, (2) the substantial eccentricities, and (3) the existence of Jovian companions with large mutual inclinations. In this work, we propose a theoretical model that satisfies these requirements without invoking tidal dissipation or large primordial inclinations. Instead, tilting is facilitated by the protoplanetary disk dispersal during the late stage of planet formation, initiating a process of resonance sweeping and parametric instability. This mechanism consists of two steps. First, a nodal secular resonance excites the inclination to large values; then, once the inclination reaches a critical value, a linear eccentric instability is triggered, which detunes the resonance and ends inclination growth. The critical inclination is pushed to high values by general relativistic precession, making polar orbits an inherently post-Newtonian outcome. Our model predicts that polar, close-in sub-Neptunes coexist with cold Jupiters in low stellar obliquity orbits. apjlabb952f4
Dynamics of small grains in transitional discs Krumholz, Ireland, & Kratter, 2020
moreTransitional discs have central regions characterized by significant depletion of both dust and gas compared to younger, optically thick discs. However, gas and dust are not depleted by equal amounts: gas surface densities are typically reduced by factors of ∼100, but small dust grains are sometimes depleted by far larger factors, to the point of being undetectable. While this extreme dust depletion is often attributed to planet formation, in this paper we show that another physical mechanism is possible: expulsion of grains from the disc by radiation pressure. We explore this mechanism using 2D simulations of dust dynamics, simultaneously solving the equation of radiative transfer with the evolution equations for dust diffusion and advection under the combined effects of stellar radiation and hydrodynamic interaction with a turbulent, accreting background gas disc. We show that, in transition discs that are depleted in both gas and dust fraction by factors of ∼100-1000 compared to minimum mass Solar nebular values, and where the ratio of accretion rate to stellar luminosity is low ( M˙/L≲10−10M⊙ yr −1L−1⊙ ), radiative clearing of any remaining ∼0.5μm and larger grains is both rapid and inevitable. The process is size-dependent, with smaller grains removed fastest and larger ones persisting for longer times. Our proposed mechanism thus naturally explains the extreme depletion of small grains commonly found in transition discs. We further suggest that the dependence of this mechanism on grain size and optical properties may explain some of the unusual grain properties recently discovered in a number of transition discs. The simulation code we develop is freely available. fig12_krumholz_2020
Instabilities in multiplanet circumbinary systems Sutherland and Kratter, 2019
moreThe majority of the discovered transiting circumbinary planets are located very near the innermost stable orbits permitted, raising questions about the origins of planets in such perturbed environments. Most favoured formation scenarios invoke formation at larger distances and subsequent migration to their current locations. Disc-driven planet migration in multiplanet systems is likely to trap planets in mean motion resonances and drive planets inwards into regions of larger dynamical perturbations from the binary. We demonstrate how planet-planet resonances can interact with the binary through secular forcing and mean-motion resonances, driving chaos in the system. We show how this chaos will shape the architecture of circumbinary systems, with specific applications to Kepler 47 and the Pluto-Charon system, limiting maximum possible stable eccentricities and indicating what resonances are likely to exist. We are also able to constrain the minimum migration rates of resonant circumbinary planets. SutherlandKratterfig4
Slowly-growing planets trigger weaker vortices: Hammer, Kratter, & Lin, 2017
moreThe presence of a giant planet in a low-viscosity disc can create a gap edge in the disc’s radial density profile sharp enough to excite the Rossby wave instability. This instability may evolve into dust-trapping vortices that might explain the `banana-shaped’ features in recently observed asymmetric transition discs with inner cavities. Previous hydrodynamical simulations of planet-induced vortices have neglected the time-scale of hundreds to thousands of orbits to grow a massive planet to Jupiter size. In this work, we study the effect of a giant planet’s runaway growth time-scale on the lifetime and characteristics of the resulting vortex. For two different planet masses (1 and 5 Jupiter masses) and two different disc viscosities (α = 3 × 10-4 and 3 × 10-5), we compare the vortices induced by planets with several different growth time-scales between 10 and 4000 planet orbits. In general, we find that slowly-growing planets create significantly weaker vortices with lifetimes and surface densities reduced by more than 50 per cent. For the higher disc viscosity, the longest growth time-scales in our study inhibit vortex formation altogether. Additionally, slowly-growing planets produce vortices that are up to twice as elongated, with azimuthal extents well above 180° in some cases. These unique, elongated vortices likely create a distinct signature in the dust observations that differentiates them from the more concentrated vortices that correspond to planets with faster growth time-scales. Lastly, we find that the low viscosities necessary for vortex formation likely prevent planets from growing quickly enough to trigger the instability in self-consistent models.
Debris in the Pluto-Charon System: Smullen & Kratter, 2017
moreThe Pluto-Charon system has come into sharper focus following the fly by of New Horizons. We use N-body simulations to probe the unique dynamical history of this binary dwarf planet system. We follow the evolution of the debris disc that might have formed during the Charon-forming giant impact. First, we note that in-situ formation of the four circumbinary moons is extremely difficult if Charon undergoes eccentric tidal evolution. We track collisions of disc debris with Charon, estimating that hundreds to hundreds of thousands of visible craters might arise from 0.3-5 km radius bodies. New Horizons data suggesting a dearth of these small craters may place constraints on the disc properties. While tidal heating will erase some of the cratering history, both tidal and radiogenic heating may also make it possible to differentiate disc debris craters from Kuiper belt object craters. We also track the debris ejected from the Pluto-Charon system into the Solar System; while most of this debris is ultimately lost from the Solar System, a few tens of 10-30 km radius bodies could survive as a Pluto-Charon collisional family. Most are plutinos in the 3:2 resonance with Neptune, while a small number populate nearby resonances. We show that migration of the giant planets early in the Solar System’s history would not destroy this collisional family. Finally, we suggest that identification of such a family would likely need to be based on composition as they show minimal clustering in relevant orbital parameters.
Scattering in Circumbinary Systems: Smullen, Kratter, & Shannon, 2016
moreIn this work, we determine how planet-planet scattering shapes planetary systems around binaries as compared to single stars. In particular, we look for signatures that arise due to differences in dynamical evolution in binary systems. We carry out a parameter study of N-body scattering simulations for four distinct planet populations around both binary and single stars. While binarity has little influence on the final system multiplicity or orbital distribution, the presence of a binary dramatically affects the means by which planets are lost from the system. Most circumbinary planets are lost due to ejections rather than planet-planet or planet-star collisions. The most massive planet in the system tends to control the evolution. Systems similar to the only observed multiplanet circumbinary system, Kepler-47, can arise from much more tightly packed, unstable systems. Only extreme initial conditions introduce differences in the final planet populations. Thus, we suggest that any intrinsic differences in the populations are imprinted by formation.
Stability of Massive Planetary Systems: Morrison & Kratter, 2016
moreIn this paper, we explore the stability of high mass, multi-planet systems detectable by direct imaging surveys. While empirical relationships exist that predict how system stability scales with planet spacing at low masses, we show that extrapolating to super-Jupiter masses can lead to up to an order of magnitude overestimate of stability for massive, tightly packed systems. We show that at both low and high planet masses, overlapping mean-motion resonances trigger chaotic orbital evolution, which leads to system instability. We attribute some of the difference in behavior as a function of mass to the increasing importance of second order resonances at high planet–star mass ratios. We use our tailored high mass planet results to estimate the maximum number of planets that might reside in double component debris disk systems, whose gaps may indicate the presence of massive bodies.
Kepler 444 or “Marses” in a Triple Star System: Dupuy, Kratter, et al 2016
moreThis work uses precision astrometry and radial velocity data to reveal the orbital configuration of one of the most extreme planetary systems: Kepler 444. The primary star in this system hosts 5 transiting sub-earth size planets with orbital periods less than 20 days. This planetary system is orbited by a highly eccentric (e ~0.9) spectroscopic M-dwarf binary that passes within 5 AU of the primary. These stars are likely co-eval with the planetary system suggesting that these tiny planets formed in a truncated disk. In addition, the outer pair has an orbital plane that is well aligned with the orbital plane of the planets! The movie below shows how the planetary orbits *would* evolve if the planetary orbits were misaligned with the outer M-dwarf pair. The rapid precession of nodes induced by the binary corroborates the finding from the best fit orbits that the planes are aligned. Otherwise it would be unlikely to catch all 5 planets in transit.
Circumbinary Planet Dynamics: Kratter & Shannon 2014
more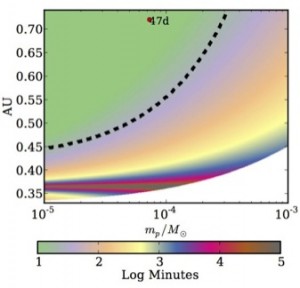
In this paper, we explore what happens to planets around one star in a binary system as that star evolves off of the main sequence and lose mass. Many planets are observed in stellar binary systems, and their frequency may be comparable to that of planetary systems around single stars. Mass loss can trigger dynamical instabilities that drive planets into chaotic orbits. This instability leads to planet-star collisions, exchange of the planet between the binary stars “star-hoppers”, and ejection of the planet from the system.Because planets often suffer close encounters with the primary on the Asymptotic Giant Branch, captures during collisions with the stellar envelope are also possible. Such capture could populate the habitable zone around white dwarfs.
The movie above shows planets in the rotating reference frame of the binary, and their zero-velocity curves. As the primary (blue) loses mass, the orbits expand. When the zero velocity curves open, transfer is allowed between the two stars, and ultimately their orbits become chaotic and the planets begin bouncing back and forth. As mass loss continues, and the curves pinch off, planets can get trapped around the secondary star.
Physical collisions and close approaches between stars play an important role in the formation of exotic stellar systems. Standard theories suggest that collisions are are, occurring only via random encounters between stars in dense clusters. We present a different formation pathway, the triple evolution dynamical instability (TEDI), in which mass loss in an evolving triple star system causes orbital instability. The subsequent chaotic orbital evolution of the stars triggers close encounters, collisions, exchanges between the stellar components, and the dynamical formation of eccentric compact binaries like Sirius. We find that the dominant type of stellar collisions is qualitatively different; most collisions involve asymptotic giant branch stars, rather than main sequence, or slightly evolved stars, which dominate collisions in globular clusters.
In the movie above, we show an example of the chaotic orbital evolution which follows mass loss. Here the blue star has recently finished losing mass to become a white dwarf star.